POWER-TO-GAS
Modern gas turbines and fuel cells (Theme 2) are efficient ways of turning gas into electrical energy. However, converting electrical energy back into gas has not yet been achieved at a commercial scale. The broad goal of this theme is to better integrate the power and gas grids by developing efficient and cost-effective methods for converting surplus electrical power into usable gas.
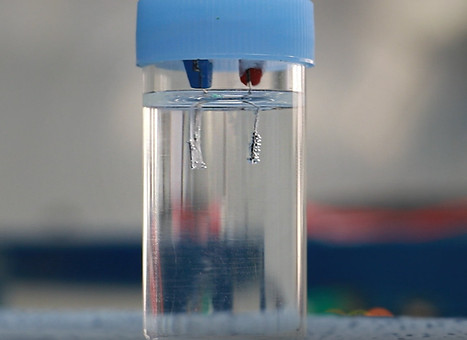
Water electrolysis using non-platinum materials
A water electrolyser is a clean process capable of converting surplus electricity generated by renewable energy sources, such as solar and wind, into H2. The H2 can be readily stored for subsequent use in fuel cell stacks (see Theme 2), as a feedstock for converting CO2 into methane (Subtheme 3.3) or converted into ammonia (Subtheme 3.4). The active component in conventional water electrolysis cathodes is typically platinum (or other platinum-group metals). Platinum is both scarce and costly relative to carbon and other transition metals, and a suitable alternative needs to be found. The subtheme will build on current research to further develop high- efficiency water electrolysers centred on non-platinum materials. Transition metals such as nickel and cobalt and, even better, carbon (a non-metal), as well as blends of these elements have exhibited similar and in some cases better activity relative to platinum-based systems. Importantly, these non-platinum systems have been shown to perform well in in alkaline and near-neutral electrolytes, including natural seawater. The advantages of such systems include high feasibility, low cost and high efficiency.
Water and/or CO2 electrolysis using reversible solid oxide cells
This sub-theme encompasses development of high efficiency and durable solid oxide cells (SOCs) for effective water and/or CO2 electrolysis, enabling storage of excess renewable electricity from renewable sources as H2. Furthermore, the electrocatalyst and cell materials will be developed for steam–CO2 co-electrolysis to convert waste CO2 (for example from coal fired power plants or ammonia plants) to syngas (Step 1), which can be used as a feedstock for further chemical synthesis including fungible liquid fuels (Step 2). The endothermic syngas generation (Step 1) and exothermic catalytic liquid fuel synthesis (Step 2) would be thermally integrated to maximise the efficiency of the overall process. This technology is of particular interest for large scale renewable energy storage and export in regions with high solar, wind or tidal power resources as well as sources of CO2. - for example, the Pilbara in Western Australia with plenty of solar energy available and the presence of industrial establishments producing large amount of CO2. Besides using CO2 from cement, fertiliser and power plants, the process can further be combined with emerging atmospheric CO2 capture technology. While the costs of atmospheric CO2 capture technology are currently high, it is not location specific and it does not depend on the specific emission source, enabling CO2 re-use to close the carbon cycle. Round-trip efficiencies in excess of 70% have been projected.
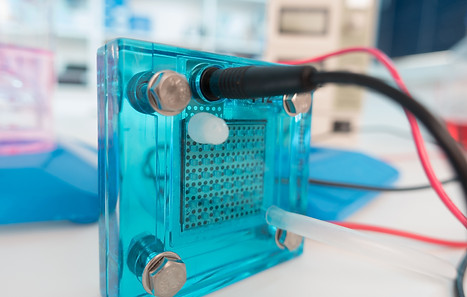
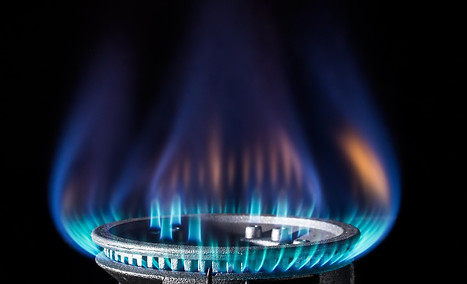
Solar synthesis of methane from H2 and waste CO2
Converting CO2 into a gaseous fuel such as methane is a means by which energy can be stored in a chemical form for later use. When the CO2 feedstock originates from the waste gas stream of an industrial process, there is the added advantage of reducing its release into the atmosphere as a greenhouse gas. Using catalytic methanation, H2 can be reacted with waste CO2 from such sources to produce methane. This can be performed via photothermal technology, with solar heating as the main driving force. With this approach, CO2-rich flue gasses can be converted into a valuable fuel using sunlight. The resulting natural gas (NG) product can be integrated into the existing natural gas network for transportation and storage. A key advantage of the photothermal methanation technology is its feasibility, since it relies on conventional thermal catalysts, which are well-established, scalable and effective. Although already well-developed for thermal-only systems, catalysts for photothermal systems must also be proficient at absorbing incident light. CIs Amal, Scott and Aguey-Zinsou have recently begun designing catalysts, based on transition metal materials, which are highly active (and selective) for CO2 methanation, as well as being capable of absorbing a broad portion of the solar spectrum. While catalyst development remains ongoing, the next phase of the work will involve adapting the catalysts to a larger-scale solar furnace (where temperatures of up to 700°C can be achieved) for the specific purpose of NG production. Integrating high temperature catalytic processes with solar thermal energy requires optimisation of solar field layouts to match the requirements of the chemical process. Additionally, thermal management of the concentrated heat and daily solar cycle present unique challenges. On-sun experimental work is critical to informing process models of the catalytic system and can be achieved at the CSRIO solar thermal facility from 10 to 1000 kWt.
Ammonia synthesis by electrochemical route
Worldwide, around 200 Mt of ammonia is produced annually, mainly for fertilisers and explosives. Ammonia is produced using the very energy-intensive Haber–Bosch process, which requires >200 bar pressure and temperatures above 450°C. Natural gas, coal and fuel oil are the fuel feedstocks used for the production of ammonia, contributing globally to over 1% of energy-related CO2 emissions and 2% of fossils fuel use. There is a significant push from the ammonia production industry to lower its energy intensity and decarbonise its processes by using renewable energy sources to produce ammonia. Owing to its high hydrogen content, liquid state under mild pressure, and existing storage and transportation regulations, ammonia may also be used as an energy vector or transport medium for hydrogen (see Subtheme 2.3). We plan to synthesise ammonia through an electrochemical route by directly coupling a renewable energy source with an ammonia synthesis reactor, then separating from unused reactants by liquefying under near ambient conditions. This is a low pressure (~10 bar) operation, and could reduce the energy input by >25% and carbon footprint by >90% compared with the conventional Haber–Bosch process. The proposed work aims to develop high performance catalysts tailored for ammonia synthesis reactions, and design the interface to maximise reaction sites, thereby increasing the conversion and ammonia synthesis rates beyond their current levels of 10–9–10–8 mol/cm2/s, which are too low to be commercially viable.
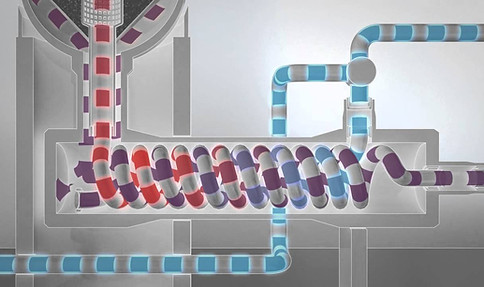
THEME LEADERSHIP
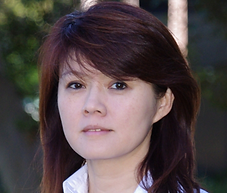